previous Municipal Solid Waste: Pelletised forms
4. Miscellaneous Waste-Derived Solid Fuels
4.1 Introduction
4.3.2 Electricity generation from wood waste fuel
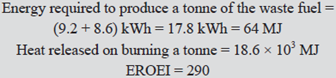
This is a remarkably high EROEI: it is highly unlikely that it is matched by any crude oil produced in the early 21st Century (although values higher than that were possible for crude oil in the 1950s and further back). It is of course based only on one set of data nor does the author wish to argue from the particular to the general. Even so the conclusion that a high EROEI can be expected from well managed production of cotton waste fuels is reasonable. EROEI is an energy quotient and attempts to express it in a financial paradigm are unsound. That being understood, the financial basis of cotton waste fuel usage is strengthened by the fact that carbon credits are generated and this is relevant to the overall viability.
4.4.4 Further examples

4. Miscellaneous Waste-Derived Solid Fuels
4.1 Introduction
‘Waste-derived solid fuels’ are legion and a revival of interest in them is expected as greenhouse gas emissions and depletion of conventional fuels continue to loom so large in world affairs. Some of the most important ones will be discussed in turn in this chapter, starting with tyre waste.
4.2 Scrap tyres
4.2.1 Preamble
The US produces hundreds of millions of scrap tyres in a single year and the UK tens of millions. Both the natural latex and any incorporated polymer are combustible and calorific values are high, sometimes in the neighbourhood of 40 MJ kg-1. It is widely known that when tyres are burnt, either simply to destroy them by incineration with no return on the heat or in fuel applications, the high volatiles content of the constituent materials makes for smoky burning as noted in an earlier chapter. There is therefore a need for excess air and close attention to particulate release. Even so tyre-derived fuel (TDF) usage is major in many countries. Also, there is co-firing of tyre waste with conventional fuel as there is of RDF with conventional fuel. Waste tyres, like RDF derived from MSW, are partially carbon-neutral. The latex part is carbon-neutral but not of course any polymer within a tyre manufactured from petrochemicals.
4.2.2 Selected examples of TDF usage
By TDF usage in this section we mean TDF alone: co-firing will be discussed subsequently. The information is in tabular form below and is followed by comments.
Reference
|
Details
|
[1] Fact Sheet, April 2005 Portland Cement Association, Skokie IL.
|
About 45 Portland cement production facilities in the US using TDF fuel at the present time.
|
[2] http://www.cmsenergy.com/content.aspx?id=288%7CNorth%20America
|
Electricity generation in Sterling CT at 31 MW level with TDF. Electricity sold for general distribution.
|
[3] http://www.accessmylibrary.com/coms2/summary_0286-119456_ITM
|
Electricity generation in the English midlands at 25 MW level with TDF as sole fuel.
|
[4] http://www.betalabservices.com/renewable-carbon/tire-derived-fuels.html
|
26 million scrap tyres used as fuel each year in the US pulp and paper industry. Shredding of the tyres necessary.
|
[5] http://www.lakincorp.com/products/tdf.html |
> 10 million tyres used as fuel for industrial water heating annually in the US.
|
The manufacture of Portland cement requires kiln temperatures as high as 1400°C and that TDF should have found application is not surprising. There is the bonus that some of the steel belting in the tyres contributes to the iron content of the product. It is recorded in [1] that Japan uses about 20 million tonnes of TDF in cement kilns annually. Many other countries including Germany, the Netherlands and Australia use TDF in cement kilns. The electricity utility at Sterling CT uses either whole or shredded tyres and is a ‘dedicated TDF-to-energy’ facility. The only other such facility in the US at the present time is the Modesto plant in Westley CA, which produces at 14MW.
In the pulp and paper industry (row four) shredding is required before tyres can be used as waste because the steel content of the tyres would otherwise cause slag formation in an unacceptably high degree.
4.2.3 Other factors in the use of TDF
The topic of TDF-coal co-firing was anticipated in the previous chapter; reference [16]([6] Giere R., LaFree S.T., Carleton L.E., Tishmak J.K. ‘Environmental impact of energy recovery from waste tyres’ Geological Society, London Special Publications 2004 236 475-498) therein, contents of which were summarised in one of the tables, was concerned with firing of waste-derived fuels including RDF with bituminous coal. As we have seen previously TDF has a calorific value if anything a little higher than that of a typical bituminous coal (although it would of course be possible to select a particular TDF and a particular coal such that the reverse was true). Hence where RDF is substituted for a bituminous coal it is on approximately a one-to-one weight basis. Reference [6]([6] Giere R., LaFree S.T., Carleton L.E., Tishmak J.K. ‘Environmental impact of energy recovery from waste tyres’ Geological Society, London Special Publications 2004 236 475-498) provides calorific values for fourteen examples of TDF, and they range from 29.2 to 37.9 MJ kg-1. The mean of those is 33.6 MJ kg-1. By way of a single illustrative example, this is about 10% higher than the value for a typical Pittsburgh coal. In countries including Turkey where there is much lignite – low rank coal having a calorific value as fired of 15 to 20 MJ kg-1 – there is some interest in TDF-lignite co-firing in which case the TDF will be by far the superior fuel in terms of calorific value.
Much of the research activity into coal-RDF co-firing is into emissions; when it is proposed to introduce co-firing limits in SO2 and NOx releases, set by regulatory bodies, have to be complied with. Reference [6] also gives sulphur contents for each of the TDF fuels and these range from 1.19 to 1.85%. This sulphur content in a coal would attract a penalty in pricing, and a disadvantage of TDF is its inevitably high sulphur content. It arises of course from vulcanisation in the manufacture.
4.3 Wood waste
4.3.1 Preamble
Wood is an important fuel in these times, as indeed it was over 100 years ago. It was surprisingly late – circa 1890 – that wood fuel usage in the US was exceeded by coal usage. A distinction has to be made between wood grown as fuel and wood waste diverted to fuel use. There has been an increase in the former by way of the ‘short rotation coppice’ and the like over the last few years. This book is however concerned only with the latter, wood waste put to use in combustion plant. The basis of the carbon neutrality of wood and the results of coal-wood co-firing were explained in a previous chapter. There are many forms of wood waste. Obvious ones are sawdust and shavings. Long before the current trend towards use of wood fuels there was use of wood as a supplementary fuel in power generation in those parts of the US with a major furniture industry. Teak products are a manufactured on a large scale in Thailand and many a tourist to that country has departed with a souvenir made of teak. Again, before wood fuels became prevalent teak waste found fuel application in Thailand. Increasingly important at the present time is the use of forest thinnings as a fuel. Woody debris from the forest floor is sometimes removed as a forest fire control measure, and if such debris can be put to fuel use in steam raising or whatever so much the better. It is of course carbon neutral, and in those terms there is a further benefit from fuel use. If wood waste of any sort is taken to a landfill it will, after time of the order of a few years, start to release methane as a decomposition product. Methane is of course a much more powerful greenhouse gas than carbon dioxide.
Seasoned wood has a calorific value of about 17 MJ kg-1. One performance criterion for a fuel is how many times its own weight of saturated steam at 1 bar it can raise. The comment was made in an earlier chapter that citrus peel can only raise just over its own weight of steam in that condition. This point is examined for wood waste in the boxed area below.
From [7]([7] Perry’s Chemical Engineer’s Handbook, McGraw-Hill NY, any available edition.) or equivalent:
specific enthalpy of liquid water at 25°C = 104.8 kJ kg-1 specific enthalpy of water vapour at 100°C = 2675.8 kJ kg-1 heat required to convert 1 kg of liquid water at 25°C steam at 100oC = (2675.8 – 104.8) × 10-3 MJ = 2.6 MJ weight of steam in this condition which could be raised by 1 kg of wood = (17/2.6) kg = 6.5 kg. |
We focus on the US for facts and figures on this. The 2005 figure for electricity production in the USA from biomass waste is 54160 GWh [8]([8] http://www.ecoleaf.com/green_energy/biomasspower.html), which converts to a generation rate of ≈ 6000 MW. The consumption of electricity in the US in 2005 was 3816000 GWh, so the percentage provided by biomass waste is:
(54160/3816000) × 100 = 1.4%
Note that targets in the US for electricity from renewable sources is 12%. Even if the calculated above figure for 2005 has doubled by the time this is being written it will fall well short of 12%. If energy crops, distinguished from wood waste by the fact that they are grown expressly for burning, are factored in the gap will close to some extent. Even then isothermal methods of making electricity, including wind farms, will have a major role to play.
4.4 Selected cellulosic wastes other than wood and paper
Wood waste has been considered in this chapter and waste paper received significant attention in Chapter 1. There are many other cellulosic waste materials, and what distinguishes these from wood is the absence of lignin, hence the perhaps preferable term ‘lignocellulosic’ for wood. Some other cellulosic wastes which have found fuel use will be discussed in this section.
4.4.1 Bagasse
Bagasse is sugar cane residue, consequently occurring in such places as Fiji and northern New South Wales. It is used on site as a fuel for the sugar mills and has a significant propensity to self-heating which has been the subject of investigations by the present author [9]([9] Raj S.C., Jones J.C. 'Self-heating in the oxidation of natural materials from Fiji' New Zealand Journal of Technology 3 199-203 (1989).) amongst others. Bagasse does however sometimes find fuel use external to the scene of its production. It is a fibrous material, of suitable consistency for fuel use. It is better than raw MSW for fuel use, being of more uniform composition and much lower in ash. It is composed of cellulose and hemi-cellulose and its calorific value is around 15 MJ kg-1.
In Brazil there are proposals for electricity generation from bagasse [10]([10] http://www.tambang.org/brazil-turning-to-sugarcane-bagasse-power-generation-to-reduce-itsdependence-on-hydropower.html). This will ease the present heavy dependence of Brazil on hydroelectric power. A case for using bagasse as fuel for thermal generation of electricity will presumably have to be made on the basis of its carbon neutrality, as Brazil is a major oil producer with extensive refining capacity so heavy residual fuel oil will be the obvious choice for electricity production.
Bagasse can be made into briquettes or into pellets for combustion use; there is significant interest in the former in Kenya [11]([11] http://stoves.bioenergylists.org/stovesdoc/Karstad/canecoal/vpnchemelil.html). When pellets made from bagasse originating in Brazil and in Cuba were studied on a comparative basis [12]([12] Erlich C., Ohman M., Bjonbom E., Fransson T.H. ‘Thermochemical properties of sugar canebagasse pellets’ Fuel 84 569-575 (2005)) the most obvious difference was ash content: 1.5% for the Cuban and 5.5% for the Brazilian.
4.4.2 Rice husks (a.k.a. rice hulls)
The table below gives details of some combustion applications of rice husks.
Reference
|
Details
|
[13] http://www.hedon.info/docs/BP48-14-Assureira.pdf |
Proposals for expansion of rice husks as fuel in Peru.
|
[14] http://www.lookatvietnam.com/2010/01/rice-husks-may-fuel-mekong-delta.html |
Proposals for electricity generation in Vietnam with rice husks as fuel.
|
[17] http://www.sabmiller.com/index.asp?pageid=1128 |
Significant use of rice husks as a boiler fuel throughout India.
|
[18] http://www.reein.org/publications/biomass/elec_pow_gen_ricehusk.htm |
Feasibility study into power generation at up to 6 MW in Bangladesh with rice husks as fuel.
|
[19] http://cdmdna.emb.gov.ph/cdm/public/cdm-ph-potential.php?main=cdmph&sub=potential
|
About 3 million tonnes per year of rice husks produced in the Philippines.
|
Peru (first row of the table) produces rice husks in annual quantities of the order of megatonnes. Traditionally some has been burnt directly in the brick industry and the remainder disposed of in ways which would not be acceptable in some countries, for example simply by burning out of doors or by dumping in a river. Consequently there have been endeavours to introduce fuel use of rice husks in Peru. The need for responsible disposal of rice husks makes them a fuel of negative financial value, a term commonly encountered in discussion of waste materials as fuels. This simply means that their disposal incurs a cost. However, the negative financial value can sometimes be offset by carbon credits generated if a fuel such as rice husks, which is carbon-neutral, is substituted for a fossil fuel.
It might at first consideration be surprising that in Vietnam (row two) rice husks would be pressed into service as a fuel as the country has large amounts of oil. What it does not have is an adequate refining and distribution infra-structure. The first refinery in Vietnam began operations as recently as 2009 [15]([15] http://www.bloomberg.com/apps/news?pid=20601087&sid=ao32X_VDkbAo&refer=home) and its capacity – about 150000 barrels per day – therefore represents the entire refining capability of Vietnam at the present time. There are similar proposals for Thailand [16]([16] http://fletcher.tufts.edu/ierp/ideas/pdfs/issue1/SasakiShotaroformat.pdf), with Japanese input. In spite of the instability of its regime, Thailand does attract Japanese investors at present. That there is rice husk usage in the Indian subcontinent is not surprising: rows three and four of the table give examples. Some of the rice husks produced in the Philippines become fuel and there are measures in place to increase fuel usage to the elimination of existing highly crude methods of disposal. The cellulosic fuel for which the Philippines is particularly noted is however coconut waste. A World Bank study has indicated that 20 MW of power could be generated in the Philippines from coconut waste fuel [19]. Reference [20]([20] https://www.cia.gov/library/publications/the-world-factbook/print/rp.html) gives the 2005 electricity consumption of the Philippines as 53.67 billion kW hours. Converting to a rate of generation:
[53.67 × 1012 W hour/(365 × 24) hour] × 10-6 = 6000 MW
which is well above what, according to the World Bank study referred to, could be produced from coconut waste.
4.4.3 Cotton waste
There has for a long time been interest in cotton waste as a fuel in countries including Paraguay. There is current interest in the US of cotton waste fuels originating in states including LA, NC and AZ. It is reported in [22]([22] http://deltafarmpress.com/mag/farming_fuel_feed_cotton/) that technologies for pelletising cotton waste are advancing. Blending of the cotton waste with a small proportion of cottonseed oil raises the calorific value of the pellets to 21 MJ kg-1, higher than that of wood (except for a few woods exceptionally high in resin) with the bonus that the oil acts as a binder.
The matter of the EROEI of fuels has previously been raised in this book, and information relevant to that for a particular cotton waste fuel originating in Arizona is given in [23]([23] Coates W. ‘Using cotton plant residue to produce briquettes’ Biomass and Bioenergy 18 201-2208 (2000)). The following is taken from [23]:
Energy required to harvest the stalks averaged 9.2 kWh/t (12.1 hp-hr/ton) for the baling system, and 8.6
kWh/t (11.4 hp-hr/ton) for chopping and moulding. Specific energy of the harvested stalks averaged
18.6 MJ/kg (7983 btu/lb)
and these data are examined in the shaded area below.kWh/t (11.4 hp-hr/ton) for chopping and moulding. Specific energy of the harvested stalks averaged
18.6 MJ/kg (7983 btu/lb)
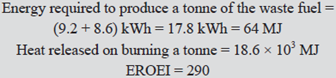
This is a remarkably high EROEI: it is highly unlikely that it is matched by any crude oil produced in the early 21st Century (although values higher than that were possible for crude oil in the 1950s and further back). It is of course based only on one set of data nor does the author wish to argue from the particular to the general. Even so the conclusion that a high EROEI can be expected from well managed production of cotton waste fuels is reasonable. EROEI is an energy quotient and attempts to express it in a financial paradigm are unsound. That being understood, the financial basis of cotton waste fuel usage is strengthened by the fact that carbon credits are generated and this is relevant to the overall viability.
We conclude this discussion of cotton waste with a reference [24]([24] Zhiao S., Shen J., Jin B., Wei L. ‘Combustion characteristics of cotton stalk in FBC’ Biomass and Bioenergy – in press.) to a very recent study of cotton stalk combustion in a fluidised bed. Cotton stalk fuel was admitted at 35 kg hour-1 to the bed, which produced 0.2 MW of heat. In the limit of adiabatic conditions the calorific value of the fuel can be calculated as:
0.2 × 106 J s-1/(35/3600) kg s-1 = 20.6 MJ kg-1
which since some heat losses must in fact have occurred is an underestimate of the calorific value.4.4.4 Further examples
The cellulosic fuels selected for discussion in this text have been wood, bagasse, rice husks and cotton wastes. There are of course very many other examples : amongst the better known are straw, peanut shells and coconut waste. Reference [25]([25] http://www.currentresults.com/Environment-Facts/Plants-Animals/number-species.php) gives a value of about 300000 for the total known number of plant species, and according to another source [26]([26] http://www.scienceclarified.com/Ca-Ch/Cellulose.html) cellulose is the most abundant organic substance on planet earth.
4.5. Solid refinery waste
4.5.1 Introduction
The world consumes about 80 million barrels of crude oil per day. It is generally held that the refining capacity of the world is somewhat larger, that is, that the petroleum industry internationally is overcapitalised with refineries. There are a number of factors relevant to this. For example, Japan is very heavily capitalised with refineries, and the entire refining capability of that country is directed at imported oil. As pointed out in an earlier chapter, Vietnam has large amounts of crude oil but only one refinery which came into operation in 2009. It was only about two years before this is being written that the first new refinery in the US since 1976 came into being and even that is intended to receive oil from Mexico! World oil production in 1976 was 57 million barrels per day, and until recently the US was able to accommodate the increases by expansion of existing refineries. If refining is on a huge scale so is the production of refinery waste. Thermal processing of such wastes is the topic of this section.
4.5.2 Refinery sludge
This comprises compounds of about C15→30 taking in therefore asphaltenes. These are the compounds which in upstream operations with crude oil can cause difficulties with flow in pipes by forming a solid matrix within the oil. This happens if the temperature goes below the pour point. Compounds in the C15→30 range form a sludge which accumulates at the bottom of storage tanks. It requires disposal and if any saleable hydrocarbons can be made from it so much the better. The Chevron Refinery in Richmond CA salvages about a million barrels a year of useable product from such waste [28]([28] http://www.chevron.com/products/sitelets/richmond/safety/waste_management.aspx) by processes including cracking and fractionation. Otherwise, incineration with return on the heat might be possible. Further examples of such treatment of refinery sludge are given in the table below, which is followed by comments.
Reference
|
Details
|
[29] Kuriakose A.P., Manjooran S.K.B. ‘Utilisation of refinery sludge for lighter oils and industrial bitumen’ Energy and Fuels 8 788-792 (1994)
|
Sludge from a refinery in India. The lighter part distilled off and found to be suitable for blending with diesel. Indifferent results when the heavier part was processed to make a hard bitumen.
|
[30] Sankran S., Pandley S., Sumathy K. ‘Experimental investigation on waste heat recovery by refinery oil sludge incineration using fluidised-bed technique’ Journal of Environmental Science and Health A33 829 – 845 (1998)
|
Fluidised bed combustion of refinery sludge, also in India. Some samples of the sludge high in moisture through having stood for a long time. This necessitated mixing of different sludges before admittance to the bed.
|
[31] Liu J., Jiang X., Zhou L., Wang H., Han X. ‘Co-firing of oil sludge with coal-water slurry in an industrial internal circulating fluidised bed boiler’ Journal of Hazardous Materials 167 817-823 (2009)
|
Co-firing of refinery sludge, produced from tank cleaning, with coal-water slurry in a fluidised bed. The sludge the primary fuel – see comments below.
|
[32] http://www.solerox.com/images/matrix_4pp_brochure.pdf |
‘Matrix Thermal Systems’ (MTS): refinery residue mixed with a non-hazardous substance such as soil and then irradiated with i.r. to produce a ‘cake’ which can legally be taken to a landfill.
|
The sludge in reference [29] had accumulated over the previous 20 or more years. The lighter material from it deemed to be a suitable blendstock for diesel had a calculated cetane index – not the same as the measured cetane number but hopefully a good approximation to it – of 45 which is a very reasonable value. The fluidised bed combustion of refinery sludge was affected by water having entered the sludge as noted in the table. The highest ‘as received’ calorific value of the sludges was 36 MJ kg-1, indicating about 15% water. The work in [30] was on a pilot scale using 30 to 50 kg per hour of the sludge. On the basis of the results it is stated that the plant in full scale will take about 2 tonnes of sludge per hour. Using the calorific value for the sludge given above, the heat release rate will be:
It was shown in section 4.3.1 of this text that the raising of 1 kg of saturated steam at 1 bar from liquid water at 25°C requires 2.6 MJ. On this basis the fluidised bed combustor under consideration could raise:

Even at a large refinery 600 tonne per day would be a very significant proportion of the steam requirement. Of course, the figure is for saturated steam at 1 bar and steam at a refinery might be superheated; any diverted to electricity generation almost certainly will be. Moreover, the refinery sludge will not necessarily be available around the clock. It is difficult to see why, if that were so, low-value heavy material from the refining residue could not be substituted. The point being made is that refinery sludge as fuel could make a significant contribution to the steam requirements of a refinery.
The matter of energy-return-on-energy-invested (EROEI) of biomass fuels was raised previously in this book. A rule of thumb is that the EROEI of a gasoline is that for the crude oil from which it was obtained divided by about 1.3. The difference is due not only to fractionation but to technologies such as fluid catalytic cracking (FCC). In the UK sector of the North Sea at the present time a value of about 6 for the EROEI of a crude oil is typical, giving a value of about 4.5 for any gasoline made from it. Skilled and judicious energy auditing at a refinery might make it possible to raise that to about 5 by use of refinery sludge in steam raising. Simply to burn the sludge with no return on the heat will incur carbon credits, making it under those circumstances a ‘fuel of negative financial value’.
The application in the third row of the table look at first sight like one of those enterprises where ‘everybody wins’. The primary fuel is refinery sludge and the benefits accruing from fuel use of that have already been described. The secondary fuel is coal-water slurry. This is a way of utilising coal fines, hich otherwise are an extreme dust explosion hazard. A stable slurry can be formed from water and coal particles of median diameter ≈ 30 μm in the correct proportions. Such a slurry will flow like a heavy fuel oil.
In Europe and elsewhere refinery sludge is classified as a hazardous waste and direct landfill disposal is precluded. The technology described in the final row of the table involves blending with soil or other harmless substance to dilute the sludge to a degree where it can be taken to a landfill. This involves infrared treatment to consolidate the mixture of sludge and ‘diluent’ into moulded units of regular shape which can conveniently be handled and transported to a landfill.
4.6 Concluding remarks
All of the wastes discussed so far are capable of processing by pyrolysis or by gasification. The next chapter will give an introduction to pyrolysis and gasification, and their application to waste management will follow. Liquid hydrocarbon waste has a chapter to itself later in the book.
No comments:
Post a Comment
Please wait for approval of your comment .......