previous Examples of Waste Pyrolysis
7. Examples of Gasification of Wastes
7.1 Raw MSW
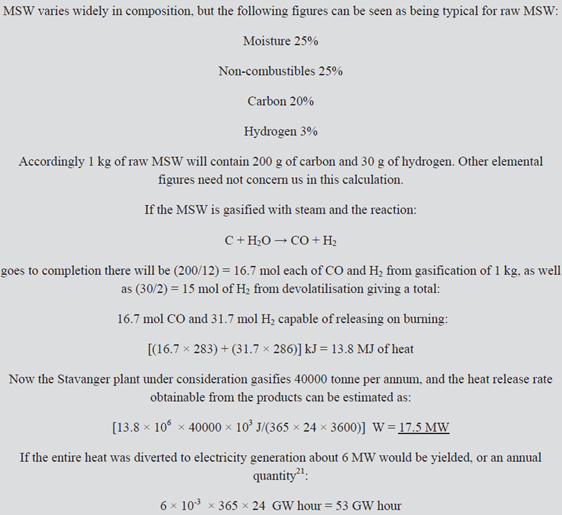
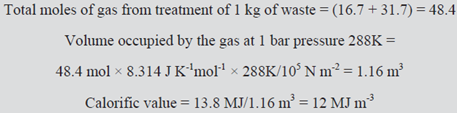
The gas in reference [4] has a calorific value of 250 BTU per cubic foot, equivalent to
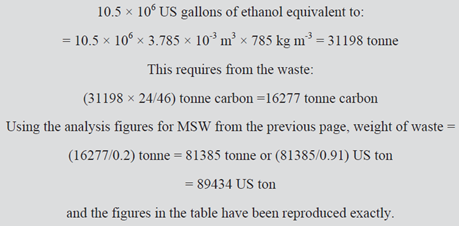
row for Lurgi gasification of lignite only and of lignite with tyre waste reveal a very marginal improvement with the co-gasification in calorific value terms, and there will of course be some carbon neutrality benefits arising from the natural rubber component of the tyres. Notwithstanding what has been said previously about ‘total gasification’, it has long been the case that Lurgi gasifiers are operated in such a way as to produce some tar by-product. For example, the Lurgi process was used in Melbourne Australia between 1956 and 1969 to make town’s gas, and there was considerable interest in the byproduct ‘Lurgi tars’. In [10] there are significant liquid by-products which have calorific vales of 38 MJ kg-1 and kinematic viscosities of 10 to 20 cSt. He or she would be an unimaginative fuel technologist who could not conceive an application for such liquids. Values for their cloud and pour points would have been a helpful addition to the information on them in [10].
The examples covered in this chapter have been diverse and will hopefully have enabled a reader to understand and interpret reports of gasification of waste. Such reports abound in the current research literature and this signifies ongoing interest and investment.
next Hydrocarbon Waste
7. Examples of Gasification of Wastes
7.1 Raw MSW
Against a background of the principles of gasification given Chapter 5 selected examples of gasification of waste will be discussed, starting with MSW. These are in the table below which is accompanied by comments.
Reference
|
Details
|
[1] http://gasification4energy.com/Gasification-Plant-Technology-Case-Studies/Energos-Gasification-Plants.php
|
Averøy, Norway. Annual processing of ≈ 16000 tonnes of MSW in addition to commercial wastes. Gaseous fuel obtained used to provide steam for a nearby factory.
|
[1]
|
Stavanger, Norway. A waste gasification plant capable of treating 40,000 tonnes per annum of MSW. Power and hot water from the gas produced.
|
[1], [3] http://www.energ.co.uk/?OBH=69&ID=19
|
Isle of Wight, England. Electricity at 2.3 MW from local MSW after gasification.
|
[4] Schilli J.W. ‘Using gasification to process MSW’ HDR Innovations 12 (4) (2004)
|
MSW compacted to a bulk density of about 1000 kg m-3. Gasification to give a product of calorific value 9 MJ m-3.
|
[5] http://www.treehugger.com/files/2008/07/municipal-waste-to-ethanol-plant-reno-nevada.php
|
Plant under construction in Nevada for synthesis gas from MSW for subsequent conversion to ethanol for fuel use. Expected production 10.5 million (US) gallons per year of ethanol from 90000 (US) tons of waste.
|
[6] http://www.gizmag.com/enerken-edmonton-waste-to-biofuels/14393/
|
Plant under construction in Edmonton, Canada to make 9.5 million gallons of ethanol annually from 110000 US tons of waste.
|
[7] Kwak T-H., Lee S., Maken S., Shin H-C., Park J-W., Yoo Y.D. ‘A study of gasification of MSW using a double inverse diffusion flame burner’ Energy and Fuels 19 2268-2272 (2005)
|
S. Korea. A three ton per day pilot gasifier yielding a gas roughly one third each in CO, CO2 and H2.
|
The plants at Averøy and at Stavanger (rows one and two) were manufactured and installed by Energos, who have plant in other countries including the UK. The information on the Stavanger plant will be used in the approximate calculations below.
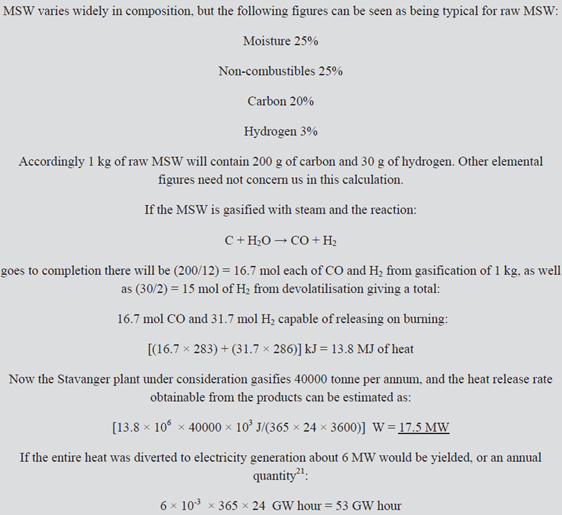
As explained in the table, at present not all of the energy is used to make power, some being used in steam generation for heating. Note also that the gas will make for a much cleaner burn than solid MSW.
The calculation can be taken a little further in the following way: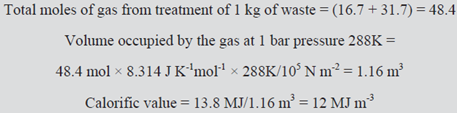
The gas in reference [4] has a calorific value of 250 BTU per cubic foot, equivalent to
250 BTU × 252 cal BTU-1 × 4.2 J cal-1/0.028 m3foot-3 = 9.5 MJ m-3
somewhat lower than that calculated above. The reason is that carbon monoxide to the exclusion of the dioxide is not quite achieved and the carbon dioxide so formed becomes a diluent.
The examples dealt with so far have produced gas for fuel use: reference [5] (fifth row) by contrast is concerned with use of the gasification product of MSW as a synthesis gas to make ethanol. An approximate mass balance will verify the production figures given in the table. This is in the shaded area below.
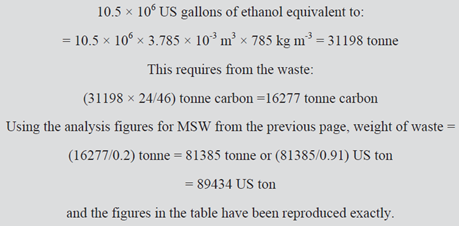
The figures in the following row for the Edmonton Canada plant correspond very closely to those for the Nevada plant so the above calculation, with its very impressive agreement with the published expected production, applies equally to the Edmonton plant.
7.2 RDF pellets as gasification feedstock
It is clear from the contents of an earlier chapter of this book that by the time MSW has become RDF pellets it has been value-added, and more is expected of it than of raw MSW. The latter might well be of negative financial value: RDF will always be of positive financial value. That for a limited number of applications RDF can replace coal is the reason for its production, and such replacement can apply not only to combustion but to gasification. It was shown in Chapter 5 how producer gas can be made by passing air through a hot bed of coal, and RDF pellets have been substituted for coal in producer gas manufacture, e.g. [7]. Air only, not air/steam, was used in the gasifier described in [7] therefore the gas was also describable as Siemens gas. A calorific value as high as 5.6 MJ m-3 was reported for the gas obtained by blowing air only through a bed of RDF, whereas Siemens has from coke as only about 4 MJ m-3 as was shown in Chapter 5. Coke has no volatiles, and Siemens gas made from a coal with a moderate volatile content, say a high-volatile bituminous, might be expected to have a somewhat higher calorific value by reason of devolatilised methane. This principle carries through to RDF pellets with their very high volatile content and given their price advantage over coal RDF pellets might on these grounds appear to be a good feedstock for producer gas manufacture. Two further points, one a plus and one a minus, can be made. The plus is that any carbon neutrality of the RDF will carry through to the producer gas. The minus is that since producer gas is made by partial combustion and involves total reaction of the organic component there being no char, the large amounts and corrosive nature of the ash from the pellets might make for difficulties. Producer gas from RDF pellets is also the theme of reference [8]([8] Morris M., Waldheim L. ‘Energy recovery from solid waste fuels using advanced gasification technology’ Waste Management 18 557-564 (1998)) which describes a gasifier taking 200 tonnes per day of RDF to make a gas of 7.4 MJ m-3. Analysis figures for the gas are given and methane is 6.5% molar basis whilst C2+ hydrocarbons are as high as 4.9%. Very possibly the higher hydrocarbons originate from tar cracking.
7.3 Co-gasification
Co-gasification might be of one waste substance with another or it might be of a waste substance with a conventional feedstock, commonly coal. Examples of the latter are given in the table below.
Reference
|
Details
|
[9] Koukouzas N., Katsiadakis A., Karlopoulos E., Kakaras E. ‘Co-gasification of solid waste and lignite - as case study for Western Macedonia’ Waste Management 28 1263-1275 (2008)
|
RDF pellet gasification with lignite and supply of the fuel gas to a steam turbine and to a gas turbine to make electricity. Lignite in a bed-moist state, having a lower calorific value than the RDF pellets.
|
[10] Straka P., Bucko Z. ‘Co-gasification of lignite/tyre waste mixture in a moving bed’ Fuel Processing Technology 90 1202-1206 (2009)
|
Tyre waste and lignite co-gasified in steam and oxygen. Calorific value of gas produce from gasification of lignite alone and that produced by co-gasification of lignite with tyre waste respectively 12.4 and 12.8 MJ m-3.
|
[11] Velez J.F., Chejne F., Valdes C.F., Emery E.J., Londono C.A. ‘Co-gasification of Colombian coal and biomass in a fluidised bed’ Fuel 88 424-430 (2009)
|
Synthesis gas from Colombian coal co-gasified with biomass in a fluidised bed. Temperatures up to ≈ 900oC.
|
[13] http://www.dgmk.de/kohle/abstracts_velen7/Dongen_Kanaar.pdf |
Co-gasification of coal with biomass to make a gas which is partly carbon neutral. Carbon credits accruing.
|
Reference [9] (first row of the table) uses a blend of 25% by weight lignite, balance RDF pellets and a third of the electricity is generated at the steam turbine, two thirds at the gas turbine. Production of electricity is a fairly modest 30 MW. The most noteworthy feature of the results in [10] is the high calorific value of the of the gas pyrolysate, up to 40 MJ m-3 at the higher end of the pyrolysis temperature range. This is due to a high proportion of C2+ alkanes, and analyses were carried out. The density of this gas is given in [10] as 1.22 kg m-3, from which the average molecular weight is:
1.22 kg m-3/42 mol m-3 = 0.029 kg mol-1
which corresponds almost exactly to the value of 0.030 kg mol-1 for ethane C2H6. Results in the following row for Lurgi gasification of lignite only and of lignite with tyre waste reveal a very marginal improvement with the co-gasification in calorific value terms, and there will of course be some carbon neutrality benefits arising from the natural rubber component of the tyres. Notwithstanding what has been said previously about ‘total gasification’, it has long been the case that Lurgi gasifiers are operated in such a way as to produce some tar by-product. For example, the Lurgi process was used in Melbourne Australia between 1956 and 1969 to make town’s gas, and there was considerable interest in the byproduct ‘Lurgi tars’. In [10] there are significant liquid by-products which have calorific vales of 38 MJ kg-1 and kinematic viscosities of 10 to 20 cSt. He or she would be an unimaginative fuel technologist who could not conceive an application for such liquids. Values for their cloud and pour points would have been a helpful addition to the information on them in [10].
Here it is not a comprehensive review or critique of the subject area, but nor is it a ‘child’s guide’. Readers at this level will, when applying the contents, need to have a professionally critical attitude and the capability to distinguish stronger R&D from weaker. Reference [11] (third row of the table) describes the co-gasification of Colombian coal with various forms of biomass. The coal is itself an asset, perhaps Colombia’s most important one: she exports about 80 million tonnes of coal per year [12] and that is three times the annual coal production of the UK. One is therefore dismayed to read in [11] that co-gasification of Colombian coal with various forms of biomass including sawdust and coffee husk produces gas having calorific values in the range 1.6 to 4.2 MJ m-3. A gas of 1.6 MJ m-3 could not sustain a flame on a burner; losses of heat and reactive intermediates to the metal burner structure would be too severe for propagation to occur. The value of 4.2 MJ m-3 is at the low limit for maintenance of a flame on a suitable burner, most likely one designed for producer gas. But that this should be the product of fluidised bed co-gasification of quality coal with biomass is a disappointing result. The product gas is presumably heavily diluted with nitrogen from air used in the gasification process.
7.4 Scenes of plasma gasification
A very brief description of this process was given in Chapter 5, and this will be backed up by a number of case studies. In reference [14]([14] Lemmens B., Elslander H., Vanderreydt I., Peys K., Diels L., Oosterlinck M., Joos M. ‘Assessment of plasma gasification of high caloric [sic] waste streams’ Waste Management 27 1562-1569 (2007)) fuel comprising carpet and textile waste having a measured carbon content of 60% underwent plasma gasification at a rate of 22 kg hour-1. Electricity consumption was in the range 82 to 112 kW. An attempt to examine these figures semi-quantitatively forms the calculation in the shaded area below.
The result obtained appears to signify an EROEI of the order of unity, possibly enhanced by carbon credits. The tests in the work under discussion were on a small scale and full assessment of the slag was not possible as there was too little of it. Such evaluation as was possible indicated that it complied with local regulations for ‘secondary building materials’. The work in reference [15]([15] Tang L., Huang H. ‘Biomass gasification using capacitively coupled RF plasma technology’ Fuel 84 2055-2063 (2005)), originating in China, was also on a small scale and was concerned with plasma gasification of biomass. Biomass feed was at 18 g hour-1. This is an order of magnitude lower than that in [14] and electricity consumption was also lower, in the range 1.6 to 2.0 kW. Gas yielded was in the range of calorific values 4.18 to 4.93 MJ m-3.
The first full-scale plasma gasifier in the US, which will be in Florida, is expected to come into operation in 2011 [16]([16] Wenner M. ‘Plasma turns garbage to gas’ Scientific American, October 2008.). The gas will be used to make electricity. It will process 1500 tons of waste per day and after its own very high electricity demand has been met there will remain 60 MW to sell to the grid.
The table below gives details of five plasma gasifiers which are either operational or under development. Comments follow the table.
Reference
|
Details
|
[17] http://www.westinghouse-plasma.com/projects/projects_under_development.php
|
St Lucie, FL. Plasma gasification of up to 3000 tons per day of waste from an existing landfill site. Up to 120 MW of electricity for sale on to customers.
|
[17]
|
New Orleans, LA. Plasma gasification of up to 2500 tons per day of waste. Electricity for supply to the grid.
|
[17]
|
Istanbul, Turkey. Plasma gasification of 144 tons per day of hazardous waste.
|
[18] http://www.epga.org/2002conference/Westinghouse.pdf |
Yoshii, Japan. Plasma gasification of 24 tonnes per day of raw MSW.
|
[18], [19] http://www.nature.com/nature/journal/v444/n7117/full/444262a.html |
Utashinai, Japan. Plasma gasification of MSW and Auto Shredder Residue (ASR) at ≈ 170 tonne per day.
|
The Florida facility (row one) will produce slag which, it has been confirmed, can be used in road construction. The facility at Yoshii (row four) is a small-scale one the electricity production from which is used entirely by a local hotel and leisure complex. ASR (row five) is the non-metallic moiety of destruction of automobiles, containing for example upholstery material, rubber and plastic. At the plant in Utashinai it is fired with coke and there is little if any electricity remaining for sale after the plant’s own requirements have been met. One difficulty is that Utashinai has a low population making waste supply to the plant limited and at times uncertain.
7.5 Concluding remarksThe examples covered in this chapter have been diverse and will hopefully have enabled a reader to understand and interpret reports of gasification of waste. Such reports abound in the current research literature and this signifies ongoing interest and investment.
next Hydrocarbon Waste
No comments:
Post a Comment
Please wait for approval of your comment .......